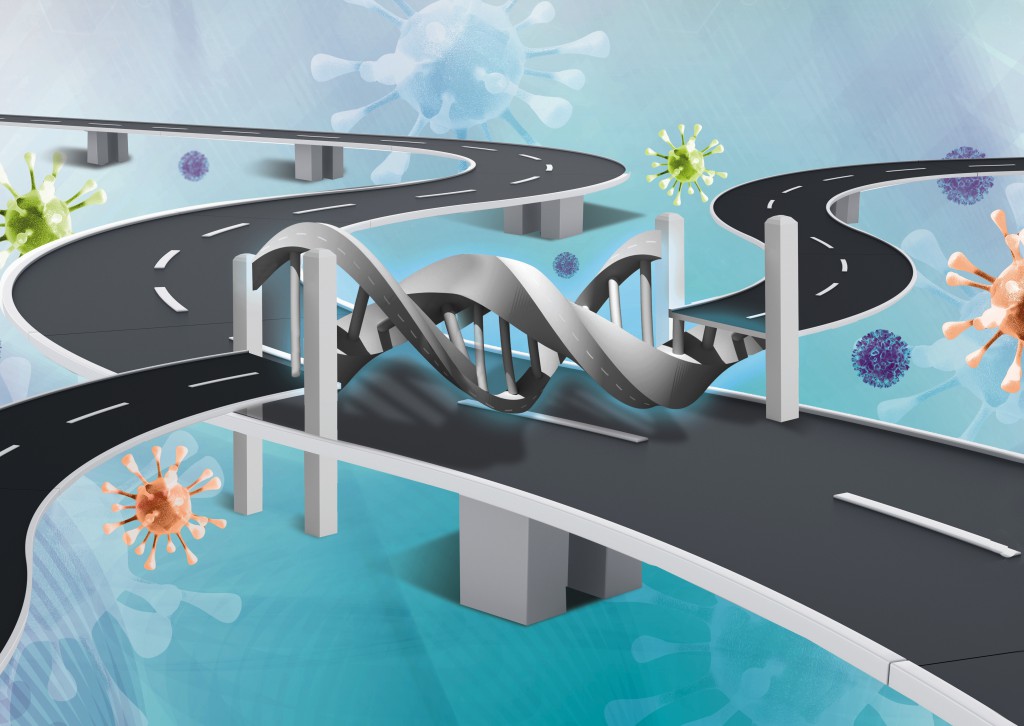
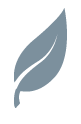
Gene Therapy’s Road to Redemption
Pediatrics Nationwide | Spring/Summer 2014
By Kelli Whitlock Burton
During the first few weeks of September in 1999, a 36-year-old air traffic controller from South Dakota and a 15-year-old high school student from Ohio checked into a hospital in Columbus, participants in the world’s first gene therapy trial to treat muscular dystrophy. Each received copies of a gene engineered in the lab to mimic the function of one of their own genes that wasn’t working correctly. The new genes were packaged neatly inside a viral vector that was injected into a muscle in the top of one foot.
The entire process took only 30 minutes but represented nearly 30 years of work by scientists such as Jerry Mendell, MD, the lead investigator on the clinical trial who was then the chair of neurology at The Ohio State University. Dr. Mendell had spent his career learning the finer points of how the neuromuscular disorder laid waste to muscle cells throughout the body. He and others had studied drugs that treated the symptoms of muscular dystrophy, but gene therapy aimed to do something those medications couldn’t — treat the condition’s underlying cause.
After decades of slow and uncertain progress, the field of gene therapy was finally moving forward. The National Institutes of Health approved a record number of new gene therapy clinical trials in 1999, Dr. Mendell’s among them. Not only was his the first gene therapy trial for muscular dystrophy, it also was the first time this particular type of viral vector — an adeno-associated virus — had been used to deliver a gene in humans. Unfortunately, there would soon be yet another first for gene therapy.
Two weeks after Dr. Mendell began his study, an 18-year-old named Jesse Gelsinger, a participant in an unrelated trial at the University of Pennsylvania, died following an unforeseen and catastrophic reaction to the adenovirus vector used to deliver a gene for the patient’s rare metabolic disorder. Gene therapy had its first fatality.
As details slowly surfaced about Gelsinger’s death, it became increasingly apparent that a series of missteps by scientists leading the trial was at least partially to blame. The leader of the Gelsinger study was also director of a lab at the university that produced and supplied a variety of viral vectors to researchers around the country. Until investigators from the Food and Drug Administration could determine why Gelsinger died, the agency suspended all gene therapy trials linked to that lab.
Almost everything about the trials in Pennsylvania and Ohio was different: two different research teams, two different diseases, two different genes and two different viral vectors. But both vectors were made at the University of Pennsylvania. Dr. Mendell had to stop his trial. Just four weeks after Dr. Mendell’s team took its momentous steps toward treating muscular dystrophy with gene therapy, the field faced a perilous future — if it had a future at all.
FROM HUMBLE BEGINNINGS
Human gene therapy became a reality on Sept. 14, 1990, in a hospital room at the National Institutes of Health in Bethesda, Md., when a 4-year-old girl with the immune disorder adenosine deaminase (ADA) deficiency received an infusion of white blood cells engineered to contain copies of the gene she lacked. It was the moment of truth for gene therapy, a concept that first arose in the 1960s with the creation of DNA-splicing technology. The idea is simple: identify genes that malfunction and cause disease and replace them with functioning copies that will treat or even cure that disease. But as is so often the case in science, turning the idea into a reality is far more complex.
After initial success in animal studies, gene therapy met with a number of highly publicized failures in the 1990s (among them the very first trial at the NIH, which only produced temporary gene expression in the girl with ADA deficiency). It had a long list of critics, including former NIH Director Harold Varmus, who in 1995 issued a report criticizing some in the scientific community for making what he said were premature claims of gene therapy’s success. The biggest stumbling block in almost every failed trial proved to be finding a vector that would transport the engineered gene to the targeted cells safely and efficiently. The death of Jesse Gelsinger illustrated that point on an international stage.
Gelsinger enrolled in a phase I clinical trial at the University of Pennsylvania for gene therapy to treat ornithine transcarbamylase deficiency, a rare metabolic disorder that causes ammonia to amass in potentially lethal levels in the bloodstream. The teenager from Arizona was admitted to the hospital on Monday, Sept. 13, and received the treatment that morning. Later that afternoon, he had a stomach ache and spiked a fever of 104.5. He awoke Tuesday disoriented and jaundiced and by that evening, had lapsed into a coma. He died two days later, a result of multi-system organ failure.
A federal investigation cited the scientists involved in the study for violating federal policies regarding the conduct of gene therapy trials. They were banned from working on any FDA-governed human clinical trials for five years. At the time, the University of Pennsylvania was one of the primary suppliers of viral vectors for gene therapy studies around the country. The fallout impacted every scientist in the field — including Dr. Mendell.
A SEVEN-YEAR PAUSE
In the 1990s, there were a handful of scientists who knew muscular dystrophy well. Dr. Mendell was one of them. Muscular dystrophy is actually a group of more than 30 genetic diseases that weaken muscles in the arms, legs, spine and in some cases, the heart and lungs. The participants in Dr. Mendell’s 1999 trial were diagnosed with a particularly destructive form called limb girdle muscular dystrophy (LGMD) type 2D. They lacked functioning copies of the alpha-sarcoglycan gene and, along with most patients with other forms of muscular dystrophy, would likely end up in a wheelchair. The air traffic controller from South Dakota had only mild symptoms so far. The teenager could barely walk.
Dr. Mendell had investigated other treatment options, but he was convinced that gene therapy offered the best shot at a successful treatment for the neuromuscular disorder. The LGMD trial was his first attempt at human gene therapy for muscular dystrophy. When the NIH halted his and other gene therapy trials at the end of 1999, Dr. Mendell was left with the frustrating task of delivering the news to the two participants, who saw the trial as their best chance at treatment.
“They were just devastated,” Dr. Mendell recalls. “We all were.”
It was seven years before that clinical trial would resume. During that time, Dr. Mendell joined the faculty in The Research Institute at Nationwide Children’s Hospital as director of the Center for Gene Therapy. His first aim was to make sure he would never again have to rely on an external lab for the vectors he needed for his studies. With support from hospital leadership, Dr. Mendell oversaw the development of a $2 million vector-manufacturing facility. And in summer 2007, Dr. Mendell and his team re-launched the LGMD trial, this time using a homegrown adeno-associated viral vector.
“Being able to do that was liberating,” Dr. Mendell says. “One thing we’ve learned from our past experiences is that you must work with a vector-manufacturing facility that is just as precise as you will be as the principal investigator of the study. It was the only way we could oversee quality control and it was imperative for our studies to continue.”
FINDING THE RIGHT VECTOR
Identifying the genes that cause disease and engineering healthy copies in the lab are not the challenges they once were, thanks to advances in genomics and sequencing technologies. But gene delivery remains a sticking point. To get the engineered genes, called transgenes, into targeted cells, scientists have used both nonviral vectors, such as liposomes and naked DNA, and viral vectors, including the adeno-associated virus Dr. Mendell has used in all of his gene therapy trials to date. Other viral vectors include retroviruses and herpes simplex virus. Adenovirus, the vector used in the ill-fated University of Pennsylvania study, has largely fallen out of favor.
Viruses exist for one purpose: to get inside cells and release their DNA. That, naturally, makes them a perfect vehicle for gene delivery. They are especially effective in gene therapy delivered ex vivo. In this case, a scientist draws some of a patient’s own cells, places them in cell culture, and injects them with the gene of interest, also called the transgene. Gene expression is confirmed and the cells are returned to the patient.
Not all diseases are good candidates for an ex vivo approach. In most cases, the cells that lack a functioning gene cannot be removed or collected outside the body. To get the gene inside targeted cells in these conditions, scientists first package the gene inside a viral vector and then either inject it directly into the affected tissue or deliver it through the circulation, called vascular delivery. In 2008, a team led by Brian Kaspar, PhD, a principal investigator in the Center for Gene Therapy, used vascular delivery to illustrate that a type of adenoassociated virus called AAV9 could cross the bloodbrain barrier, enabling scientists to deliver transgenes directly into the brain and cerebral spinal fluid.
The research, published in Nature Biotechnology, is the foundation behind a new strategy to treat spinal muscular atrophy (SMA) type I, the most common genetic cause of infant death. Patients with SMA lack a gene called SMN, which produces a protein vital to the health of nerve cells in the brain and spinal cord. In a phase I clinical study, which has received a new investigational drug status from the FDA and is funded by Sophia’s Cure Foundation, scientists will deliver an SMN transgene in infant patients with this devastating disease. Scientists are also conducting pre-clinical studies to see whether the AAV9-delivered SMN gene can be delivered through the cerebrospinal fluid. That work is funded by the Families of SMA and the National Institute of Neurological Disease and Stroke.
BATTLING THE IMMUNE SYSTEM
Researchers in the Center for Gene Therapy will also soon launch a new trial in LGMD using another AAV vector, rh74, a virus isolated from rhesus macaque monkeys. This adeno-associated virus was developed at Nationwide Children’s and could address another key challenge to gene therapy: immune response. Other AAV viral vectors used in gene therapy are human viruses, which means that patients undergoing therapy may already have antibodies against the virus. The immune system recognizes the virus and launches an attack, killing the virus before it can deliver the gene it carries.
“Because rh74 is a non-human primate virus, there is less likelihood that human patients will already have antibodies to the virus, which is the ideal scenario,” says Louise Rodino-Klapac, PhD, a principal investigator in the center who will lead this new trial with Dr. Mendell. “Gene expression is inhibited by pre-existing antibodies because the antibodies block the AAV virus from entering the cells.”
Having rh74 in the tool box gives scientists another vector to choose from, which history suggests will be key to the success of gene therapy. Another secret to success, Dr. Mendell notes, is knowing that there is new knowledge to be gained from every experiment — even those that fail.
Such was the case with the world’s first gene therapy trial in Duchenne muscular dystrophy (DMD), the most common form in children. Launched in spring 2007 and led by Dr. Mendell, the trial used a gene therapy product developed by scientists at the University of North Carolina to treat DMD, which is caused by a missing or defective gene that makes dystrophin, a protein vital for healthy muscle tissue. The gene that makes dystrophin is the largest known human gene, too large to package into conventional viral vectors. So the UNC scientists created minidystrophin, a smaller but functional version of the human gene.
Once a transgene is injected, it should start expressing protein within a week, with optimal expression occurring at about four to six weeks. However, when scientists examined muscle biopsies from patients in the DMD trial, they found no gene expression. The therapy had failed, but it taught the team a crucial new element about the disease.
Patients in the trial had large deletions within the gene that produces dystrophin. When the transgene was injected and made its way into muscle cells, the boys’ immune systems attacked it. The immune response was striking — and puzzling. Because transgenes are meant to replace a gene innate to humans, immune reactions to the gene itself are rare. With the aid of Christopher Walker, PhD, director of the Center for Vaccines and Immunity at Nationwide Children’s, the researchers discovered that the patients’ T cells recognized parts of the dystrophin transgene as foreign because of the patients’ own genetic deletions.
This is just one example of how the body’s immune system has repeatedly thwarted gene therapy efforts. While studies continue to identify ways to molecularly manipulate immune response, Dr. Rodino-Klapac and her colleagues have figured out how to level the playing field mechanically with plasmapheresis. Widely used to treat patients with autoimmune disorders, plasmapheresis removes blood from the body, filters out antibodies and returns the blood to the patient. The antibody loss is temporary; the body begins producing new antibodies within a few hours following the procedure.
In a study published in 2013 in Molecular Therapy, the team used plasmapheresis in a large animal model, then injected a virus packed with a micro-dystrophin gene developed to treat DMD, a slightly smaller version of the minidystrophin gene used in the original trial. When they examined the levels of micro-dystrophin gene expression in the animals, they found a 500 percent increase over gene expression in animals that did not receive plasmapheresis.
“One of the problems we are faced with moving forward is that when patients get the first treatment, their bodies will develop antibodies to the virus used to deliver the gene,” Dr. Rodino-Klapac says. “Using plasmapheresis on someone who previously received gene therapy could allow them to be treated again.”
THE JOURNEY CONTINUES
Much has been learned in the 15 years since Jesse Gelsinger died. “We learn new lessons from this work every day,” Dr. Mendell says. “You always find yourself wishing you were smarter than you were when you started, but that’s part of science. You do everything you can to protect your patients but when mistakes are made, you study them, learn from them and do your best to never repeat them.”
Gene therapy may have been around for more than five decades, but as Dr. Mendell will attest, the field is still very much in its infancy. Only three gene therapy products have been approved for use — one in Europe and two in China. The United States has yet to approve a gene therapy product, although there are many in the pipeline.The Journal of Gene Medicine estimates there are nearly 2,000 gene therapy clinical trials underway worldwide, with the vast majority in the United States.
Now, scientists also are looking at using gene technology to repair rather than replace mutated genes, silence overactive genes and retrofit patients’ own immune cells with the tools they need to recognize and kill cancer cells. New genome editing studies are examining ways to remove dysfunctional genes and replace them with corrected versions, a copy and paste maneuver that takes gene replacement a step further than just adding corrected genes. This is crucial for disorders in which the malfunctioning genes are doing something harmful, as in some blood disorders, autoimmune illnesses and some types of cancer.
New drugs take years to reach the marketplace. Gene therapy takes years just to reach human trials. The preclinical and toxicology preparatory studies for the upcoming LGMD phase I clinical trial with the new rh74 vector took two and a half years and more than $1 million human clinical trials, and the price tag and time required to get the drug ready for FDA approval will likely double. Among the most painful aspects of this work, Dr. Mendell says, is telling parents and families to be patient. Indeed, it’s something he has a hard time telling himself.
“I’ve been involved with muscular dystrophy research for 40 years and most of my contemporaries, people I’ve worked with in the past, are gone,” Dr. Mendell says. “I believe this is the best and safest approach for these patients and I am very determined to see it succeed before I’m gone, too.”